1. What are Autonomous Vehicles :
Autonomous vehicles (AVs) use technology to partially or entirely replace the human driver in navigating a vehicle from an origin to a destination while avoiding road hazards and responding to traffic conditions. The Society of Automotive Engineers (SAE) has developed a widely adopted classification system with six levels based on the level of human intervention. The U.S. National Highway Traffic Safety Administration (NHTSA) uses this classification system.
AV research started in the 1980s when universities began working on two types of AVs: one that required roadway infrastructure and one that did not. The U.S. Defense Advanced Research Projects Agency (DARPA) has held “grand challenges” testing the performance of AVs on a 150-mile off-road course. No vehicles successfully finished the 2004 Grand Challenge, but five completed the course in 2005. In 2007, six teams finished the third DARPA challenge, which consisted of a 60-mile course navigating an urban environment obeying normal traffic laws. In 2015, the University of Michigan built Mcity, the first testing facility built for autonomous vehicles. Research is conducted there into the safety, efficiency, accessibility, and commercial viability of AVs. Unmanned aircraft systems (UAS), or drones, are being deployed for commercial ventures such as last-mile package delivery, medical supply transportation, and inspection of critical infrastructure.
AVs use combinations of technologies and sensors to sense the roadway, other vehicles, and objects on and along the roadway.
2. The SAE AV classification system is broken down by level of automation:
Level 0 (No Driving Automation)
Most vehicles on the road today are Level 0: manually controlled. The human provides the “dynamic driving task” although there may be systems in place to help the driver. An example would be the emergency braking system―since it technically doesn’t “drive” the vehicle, it does not qualify as automation.
Level 2 (Partial Driving Automation)
This means advanced driver assistance systems or ADAS. The vehicle can control both steering and accelerating/decelerating. Here automation falls short of self-driving because a human sits in the driver’s seat and can take control of the car at any time. Tesla Autopilot and Cadillac (General Motors) Super Cruise systems both qualify as Level 2.
Level 3 (Conditional Driving Automation)
The jump from Level 2 to Level 3 is substantial from a technological perspective, but subtle if not negligible from a human perspective. Level 3 vehicles have “environmental detection” capabilities and can make informed decisions for themselves, such as accelerating past a slow-moving vehicle. But―they still require a human override. The driver must remain alert and ready to take control if the system is unable to execute the task.
Level 4 (High Driving Automation)
The key difference between Level 3 and Level 4 automation is that Level 4 vehicles can intervene if things go wrong or there is a system failure. In this sense, these cars do not require human interaction in most circumstances. However, a human still has the option to manually override.
Level 5 (Full Driving Automation)
Level 5 vehicles do not require human attention―the “dynamic driving task” is eliminated. Level 5 cars won’t even have steering wheels or acceleration/braking pedals. They will be free from geofencing, able to go anywhere and do anything that an experienced human driver can do. Fully autonomous cars are undergoing testing in several pockets of the world, but none are yet available to the general public.
3. Current and Projected Market
Researchers forecast that by 2025 we’ll see approximately 8 million autonomous or semi-autonomous vehicles on the road. Before merging onto roadways, self-driving cars will first have to progress through 6 levels of driver assistance technology advancements.
Market Leaders
- Waymo has tested its vehicles by driving over 20 million miles on public roads and tens of billions of miles in simulation.
- Teslas have driven over 3 billion miles in Autopilot mode since 2014.
- Other major contributors include Audi, BMW, Daimler, GM, Nissan, Volvo, Bosch, Continental, Mobileye, Valeo, Velodyne, Nvidia, Ford, as well as many other OEMs and technology companies.
Regulations, Liability, and Projected Timeline
- Regulation will directly impact the adoption of AVs. There are no national standards or guidelines for AVs, allowing states to determine their own. In 2018, Congress worked to pass the AV Start Act that would have implemented a framework for the testing, regulating, and deploying of AVs. The legislation failed to pass both houses. As of February 2020, 29 states and D.C. have enacted legislation regarding the definition of AVs, their usage, and liability, among other topics.
- Product liability laws need to assign liability properly when AV crashes occur, as highlighted by the May 2016 Tesla Model S fatality. Liability will depend on multiple factors, especially whether the vehicle was being operated appropriately to its level of automation.
- Although many researchers, OEMs, and industry experts have different projected timelines for AV market penetration and full adoption, the majority predict Level 5 AVs around 2030.
Metrics and Associated Impacts
- Congestion: Congestion is predicted to decrease, reducing fuel consumption by 0-4%. However, decreased congestion is likely to lead to increased vehicle-miles traveled (VMT), limiting the fuel consumption benefit.
- Eco-Driving: Eco-Driving, a set of practices that reduce fuel consumption, are predicted to reduce energy consumption by up to 20%. However, if AV algorithms do not prioritize efficiency, fuel efficiency may actually decrease.
- Platooning: Platooning, a train of detached vehicles that collectively travel closely together, is expected to reduce energy consumption between 3-25% depending on the number of vehicles, their separation, and vehicle characteristics.
- De-emphasized Performance: Vehicle performance, such as fast acceleration, is likely to become de-emphasized when comfort and productivity become travel priorities, potentially leading to a 5-23% reduction in fuel consumption.
- Improved Crash Avoidance: Due to the increased safety features of AVs, crashes are less likely to occur, allowing for the reduction of vehicle weight and size, decreasing fuel consumption between 5-23%.
- Vehicle Right-Sizing: The ability to match the utility of a vehicle to a given need. Vehicle right-sizing has the potential to decrease energy consumption between 21-45%, though the full benefits are only likely when paired with a ride-sharing on-demand model.
- Higher Highway Speeds: Increased highway speeds are likely due to improved safety, increasing fuel consumption by 7-30%.
- Travel Cost Reduction: AVs are predicted to reduce the cost of travel due to decreased insurance cost and cost of time due to improvements in productivity and driving comfort. These benefits could result in increased travel potentially increasing energy consumption by 4% to 60%.
- New User Groups: AVs are likely to increase VMT, especially for elderly and disabled users, and fuel consumption from new users by 2-10%.
- Changed Mobility Services: Ride-sharing on-demand business models are likely to utilize AVs due to the significant reduction of labor costs. The adoption of a ride-sharing model is estimated to reduce energy consumption by 0-20%.
- Although an accurate assessment of these interconnected impacts cannot currently be made, one study evaluated the potential impacts of four scenarios, each with unknown likelihoods. The most optimistic scenario projected a 40% decrease in total road transport energy and the most pessimistic scenario projected a 105% increase in total road transport energy.
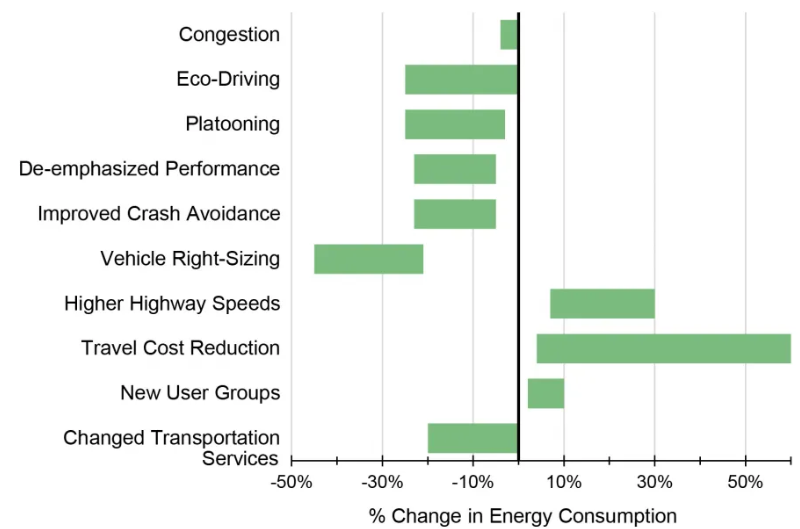